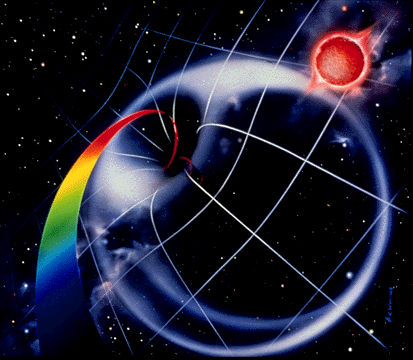
Theoretical Background
The notion of black holes originated near the end of the 18th century with the English physicist John Michell and then later by the French mathematician Pierre Simon de Laplace, who realized that if light was composed of particles, a sufficiently compact gravitating astronomical body could have such a high surface gravity that light particles (photons) could not escape from its surface, resulting in an invisible or black star.
In the early 20th century, Albert Einstein showed that no material particle could travel faster than the speed of light; therefore, neither light nor matter could escape from the black star (hence the modern name, black hole). Einstein’s General Theory of Relativity is used to study black holes, since the older theory of gravity developed by Sir Isaac Newton does not provide an adequate basis for discussing the curved space-time near them. Newtonian theory does not impose any limit on the propagation speed for material or information, which results in a theory allowing instantaneous action at a distance leading to serious violations of causality.
Event Horizon
The black hole consists of a spherical surface, called the event horizon, which separates the part of space accessible to an outside observer from the inside of the black hole from which nothing can escape. The radius of this sphere, called the Schwarzchild radius in honour of the German astrophysicist who derived the first black hole solution to Einstein’s equations in 1916, is proportional to the mass of the object that collapsed to form the black hole. For an object with mass equal to that of the sun, this radius is only about 3 km.
Werner Israel of the University of Alberta conjectured in 1967 that, once formed, a black hole can be described by a very small set of parameters which have been shown to be the total mass, the electric charge, and the angular momentum of any matter that went in to form the black hole. Thus essentially all information about the detailed structure of objects thrown into a black hole is lost.
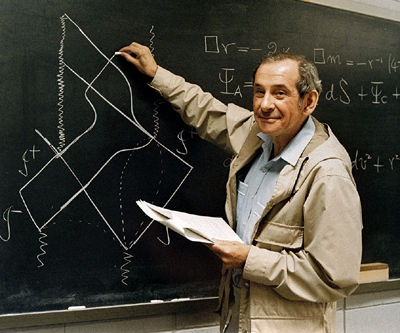
Singularity
The event horizon prevents an observer outside it from gaining any knowledge about the other significant feature of a black hole, the central singularity where gravitational physics no longer applies. It appears that most, if not all, singularities that could possibly occur are enclosed by an event horizon, leading to the conjecture of “cosmic censorship.” Thus the only observers who have any hope of investigating the singularity are those who are doomed to vanish into the singularity, without ever disclosing their findings to the rest of the universe.
Evidence for the Existence of Black Holes
The existence of black holes outside the realm of theory has been difficult to demonstrate, since to prove explicitly the existence of the event horizon that characterizes a black hole requires that the observer penetrate the event horizon, but then the information is lost since the observer cannot return. Thus the existence can only be inferred from the effects of a black hole on its surroundings or from a clear evolutionary sequence that leads to the formation of the black hole.
The theory of the later stages of the evolution of massive stars indicates that a sufficiently massive spherical star will be unable to resist its own self-gravitation forever and must eventually collapse to form a black hole. This is the basis for the contention by the University of Toronto’s C.T. Bolton that a black hole exists in Cygnus X-1. If stars can undergo large-scale mass loss during their collapse, as suggested by the work of Sun Kwok of University of Calgary and others, then many massive stars may be able to avoid collapse to a black hole.
In 1974, Stephen Hawking of Cambridge University, UK, demonstrated theoretically that a black hole radiates at a temperature inversely proportional to its mass. For a solar-mass black hole, the temperature of radiation is about 10-7 Kelvin, much below observable limits, but for a mass the size of a large mountain, the temperature is about 1011K. This theory results in the consideration of such mini black holes as sources for highly energetic cosmic radiation. Primordial black holes, much less massive than these, would probably have had time to evaporate since the beginning of the universe.
The accumulation of evidence supporting the existence of black holes indicates the possibility of two classes of these phenomena. Stellar mass black holes are of the order of a few times our sun’s mass, which emit X-rays as material falls in. Their existence is based on observations of binary stars in our own and neighbouring galaxies. The second class of black holes are the massive ones at the centre of galaxies, whose existence can be postulated by the observation of the high concentration of light distribution from these galaxies.
The existence of black holes outside the realm of theory gained support with the discovery of what appeared to be a black hole in the huge elliptical galaxy M87. One piece of evidence for a black hole would be an “accretion disk,” material spiraling into an apparent black hole like water draining out of a bathtub. On 27 February 1994, scientists using NASA’s Hubble Space Telescope discovered just such an accretion disk at the centre of M87, in the constellation Virgo, 50 million light years away from Earth. The discovery is based on velocity measurements of a whirlpool of hot gas orbiting around the black hole in the form of a disk.
That the disk was spinning was proven by observation of the Doppler effect, the familiar phenomenon noticeable when you listen to the whistle on a train as it passes. As the train approaches at high speed, the frequency of the train whistle is higher and as it travels away, its frequency gets lower. Similarly, if the light seen in a telescope is coming from an object that is approaching rapidly, its frequency gets higher, shifting toward the short wavelength end of the spectrum (a blueshift). If the object moves rapidly away, its light is redshifted. The redward and blueward shifts are discernible with a spectrograph.
Dr. Richard Harms of the Applied Research Corp. in Landover, Maryland, declared that a massive black hole may actually be a conservative explanation for what exists in M87. If it is not a black hole, it must be something even more difficult to understand and explain with present theories of astrophysics. Harms and Dr. Holland Ford, of the Space Telescope Science Institute and Johns Hopkins University, used the Faint Object Spectrograph to measure the speeds of orbiting gas on either side of the disk from regions located about 60 light years from the black hole at the centre. They calculated that the disk of hot (about 10,000 Kelvin) ionized gas is rotating at tremendous speeds around a central object that is extremely massive but extraordinarily compact — a black hole.
In 2019, scientists unveiled the first-ever direct image of a black hole after further observations of the object at the centre of M87. The picture was produced using data from a global network of eight radio telescopes acting as a single Earth-size telescope. Astronomers called the image, which shows a bright ring of debris around the event horizon, “the strongest evidence to date for the existence of supermassive black holes.”
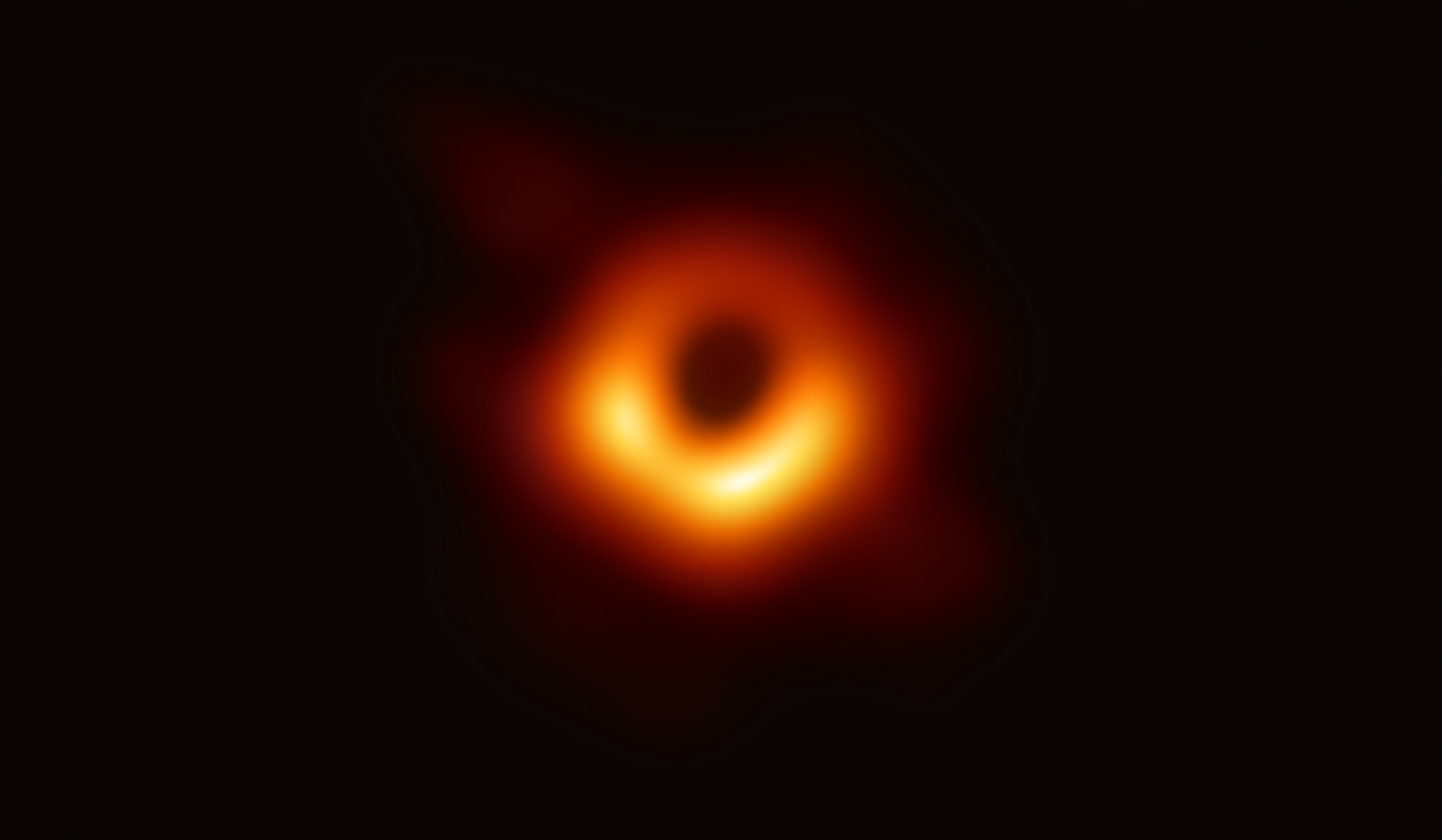
A Unified Theory of Physics
The size of a primordial black hole is such that the theories of gravitation and of quantum mechanics must both be used in the description of such objects. Thus the consideration of these objects is a step along the long-sought path to a unified theory of physics. This relationship between gravitation and quantum mechanics is now of great importance in the study of theories about the very early structure of the universe, through the Big Bang model, the inflationary model and other models of the universe. In the earliest moments of the universe, the distance scales characteristic of gravitation and quantum mechanical effects merge, so there is little chance of significant progress without a proper understanding of some unified theory of space-time.